Slideshow code developed in collaboration with ChatGPT3 at https://chat.openai.com/
Like ancient mariners, ancestors of Prochlorococcus microbes rode out to sea on exoskeleton particles | MIT News | Massachusetts Institute of Technology
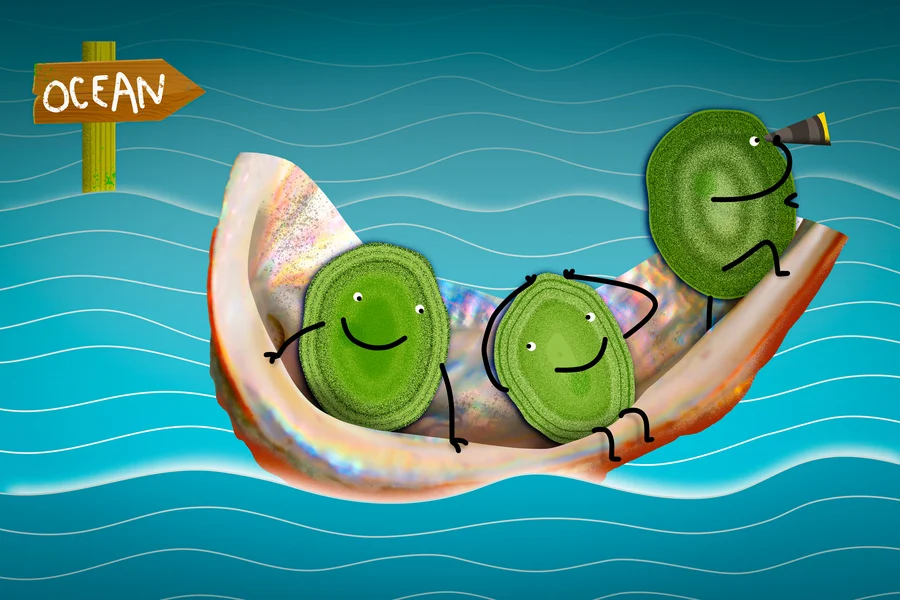
New research suggests the Prochlorococcus microbe’s ancient coastal ancestors colonized the ocean by rafting out on chitin particles.
Image: Jose-Luis Olivares/MIT
For example, it was not obvious how an abundant member of the oceanic phytoplankton, Prochlorococcus came to exist as a free-living organism, when they probably started out as organisms that lived close to the shore as biofilms on the seabed.
Prochlorococcus, a prokaryote picocyanobacterium, is the most abundant of the billions of organisms that make up what has been described as a floating forest that soaks up almost as much CO2 as the world's terrestrial forests and pumps out almost as much oxygen.
It is also the base of the food chain in the oceans.
Prochlorococcus marinusSo, how did the TOE help explain the transition from shore-based biofilm existence and free-floating oceanic existence? There had to be an intermediate stage because evolution doesn't progress in large jumps, and this was discovered by researchers in the Department of Earth, Atmospheric, and Planetary Sciences (EAPS) of the Massachusetts Institute of Technology (MIT). The prediction from the TOE was proved correct.
Prochlorococcus marinus is a species of marine cyanobacteria that is considered one of the most abundant photosynthetic organisms on Earth. It plays a vital role in the global carbon cycle and is a major contributor to oxygen production in the oceans. Prochlorococcus was discovered in 1986 by Chisholm et al. and has since been extensively studied due to its ecological significance and unique characteristics. Here are some key facts about Prochlorococcus marinus:
- Abundance and Distribution:
- Prochlorococcus marinus is found in large numbers throughout the world's oceans, primarily in the photic zone (the upper layers of the ocean where sunlight penetrates).
- It has a broad geographic distribution, ranging from tropical to temperate and polar regions.
- Prochlorococcus is often most abundant in oligotrophic (nutrient-poor) regions of the ocean.
- Morphology and Pigments:
- Prochlorococcus is a small unicellular cyanobacterium, with a diameter of around 0.6 to 0.8 micrometers.
- It lacks phycobilins, which are pigments commonly found in other cyanobacteria, and instead contains chlorophyll a and divinyl-chlorophyll a as its primary photosynthetic pigments.
- The absence of phycobilins and the presence of divinyl-chlorophyll a enable Prochlorococcus to optimize its photosynthetic efficiency in low-light conditions.
- Genomic Diversity:
- Prochlorococcus marinus exhibits genomic variation within and between its ecotypes, which is believed to contribute to its ability to adapt to different environmental conditions.
- These ecotypes are adapted to different light and nutrient conditions, allowing Prochlorococcus to occupy a wide range of ecological niches.
- Variations in the genome also reflect adaptations to different temperature regimes, salinity levels, and other environmental factors.
- Studies have revealed differences in gene content, gene expression patterns, and regulatory mechanisms among different ecotypes, highlighting their distinct metabolic strategies and ecological specialization.
- Photosynthesis and Nutrient Acquisition:
- Prochlorococcus is an autotrophic organism, meaning it synthesizes its own organic compounds using energy from sunlight through photosynthesis.
- It can carry out both oxygenic and anoxygenic photosynthesis, depending on the availability of light and nutrients.
- Prochlorococcus has evolved efficient nutrient uptake mechanisms, allowing it to scavenge trace amounts of essential elements, such as iron and phosphorus, which are limited in the oceanic environment.
- Ecological Significance:
- Prochlorococcus marinus is a key primary producer in the oceans, responsible for a significant portion of the global marine primary productivity.
- Its high abundance and rapid growth rates make it a vital contributor to carbon fixation and the cycling of nutrients in marine ecosystems.
- Prochlorococcus serves as a crucial food source for a variety of marine organisms, including zooplankton and other phytoplankton species.
- Ecological Interactions:
- Prochlorococcus marinus interacts with various organisms in the marine ecosystem, both directly and indirectly.
- It forms symbiotic associations with certain types of bacteria and viruses, which can impact its growth and survival.
- Viruses, known as bacteriophages, infect Prochlorococcus cells and play a significant role in regulating its population dynamics.
- Prochlorococcus is also preyed upon by grazers such as heterotrophic nanoflagellates and copepods, influencing its abundance and distribution.
- Environmental Responses:
- Prochlorococcus marinus has evolved strategies to respond to environmental changes and fluctuations.
- It can regulate its pigment composition and photosynthetic machinery to optimize its light absorption and utilization efficiency in response to varying light conditions.
- Prochlorococcus can adjust its cellular metabolism and gene expression patterns in response to nutrient availability, temperature, and other environmental factors.
- Role in Climate Regulation:
- Prochlorococcus marinus, as a primary producer, plays a crucial role in regulating global climate.
- Through photosynthesis, it absorbs carbon dioxide (CO2) from the atmosphere, reducing greenhouse gas concentrations and contributing to carbon sequestration in the oceans.
- Its high growth rates and abundance make it an important factor in the biological pump, which transports carbon from the surface ocean to deeper layers.
- Future Research:
- Prochlorococcus marinus continues to be an active area of research, with ongoing studies aiming to further understand its genomic diversity, ecophysiology, and ecological interactions.
- Researchers are investigating how Prochlorococcus responds to climate change and how it may influence or be influenced by changes in oceanic conditions. Advances in genomic sequencing and analysis techniques have provided insights into the metabolic capabilities, evolutionary history, and biogeography of Prochlorococcus.
References:Please note that the references provided are just a selection, and there is a vast body of research available on Prochlorococcus marinus.
- Chisholm, S.W., Frankel, S.L., Goericke, R., Olson, R.J., Palenik, B., Waterbury, J.B., & West-Johnsrud, L. (1988). Prochlorococcus marinus nov. gen. nov. sp.: An oxyphototrophic marine prokaryote containing divinyl chlorophyll a and b. Archives of Microbiology, 151(3), 155-166. (pdf)
- Partensky, F., Hess, W.R., & Vaulot, D. (1999). Prochlorococcus, a Marine Photosynthetic Prokaryote of Global Significance. Microbiology and Molecular Biology Reviews, 63(1), 106-127.
- Moore, L.R., & Chisholm, S.W. (1999). Photophysiology of the Marine Cyanobacterium Prochlorococcus: Ecotypic Differences among Cultured Isolates. Limnol. Oceanogr., 44(3), 1999, 628–638
- Coleman, M.L., et al. (2006). Genomic Islands and the Ecology and Evolution of Prochlorococcus. Science, 311(5768), 1768-1770.
- Biller, S.J., et al. (2015). Genomes of Diverse Isolates of the Marine Cyanobacterium Prochlorococcus. Scientific Data, 2, 150047.
- Behrenfeld, M.J., et al. (2006). Climate-driven Trends in Contemporary Ocean Productivity. Nature, 444(7120), 752-755.
chatGPT3 "Tell me all about Prochlorococcus marinus with references, please" [Response to user question]
Retrieved from https://chat.openai.com/
The news release from MIT, explains:
Throughout the ocean, billions upon billions of plant-like microbes make up an invisible floating forest. As they drift, the tiny organisms use sunlight to suck up carbon dioxide from the atmosphere. Collectively, these photosynthesizing plankton, or phytoplankton, absorb almost as much CO2 as the world’s terrestrial forests. A measurable fraction of their carbon-capturing muscle comes from Prochlorococcus — an emerald-tinged free-floater that is the most abundant phytoplankton in the oceans today.The MIT team's paper is published, open access, in the journal of the US National Academy of Science (PNAS):
But Prochlorococcus didn’t always inhabit open waters. Ancestors of the microbe likely stuck closer to the coasts, where nutrients were plentiful and organisms survived in communal microbial mats on the seafloor. How then did descendants of these coastal dwellers end up as the photosynthesizing powerhouses of the open oceans today?
MIT scientists believe that rafting was the key. In a new study they propose that ancestors of Prochlorococcus acquired an ability to latch onto chitin — the degraded particles of ancient exoskeletons. The microbes hitched a ride on passing flakes, using the particles as rafts to venture further out to sea. These chitin rafts may have also provided essential nutrients, fueling and sustaining the microbes along their journey.
Thus fortified, generations of microbes may have then had the opportunity to evolve new abilities to adapt to the open ocean. Eventually, they would have evolved to a point where they could jump ship and survive as the free-floating ocean dwellers that live today.
“If Prochlorococcus and other photosynthetic organisms had not colonized the ocean, we would be looking at a very different planet,” says Rogier Braakman, a research scientist in MIT’s Department of Earth, Atmospheric, and Planetary Sciences (EAPS). “It was the fact they were able to attach to these chitin rafts that enabled them to establish a foothold in an entirely new and massive part of the planet’s biosphere, in a way that changed the Earth forever.”
Braakman and his collaborators present their new “chitin raft” hypothesis, along with experiments and genetic analyses supporting the idea, in a study appearing this week in PNAS.
MIT co-authors are Giovanna Capovilla, Greg Fournier, Julia Schwartzman, Xinda Lu, Alexis Yelton, Elaina Thomas, Jack Payette, Kurt Castro, Otto Cordero, and MIT Institute Professor Sallie (Penny) Chisholm, along with colleagues from multiple institutions including the Woods Hole Oceanographic Institution.
A strange gene
Prochlorococcus is one of two main groups belonging to a class known as picocyanobacteria, which are the smallest photosynthesizing organisms on the planet. The other group is Synechococcus, a closely related microbe that can be found abundantly in ocean and freshwater systems. Both organisms make a living through photosynthesis.
But it turns out that some strains of Prochlorococcus can adopt alternative lifestyles, particularly in low-lit regions where photosynthesis is difficult to maintain. These microbes are “mixotrophic,” using a mix of other carbon-capturing strategies to grow.
Researchers in Chisholm’s lab were looking for signs of mixotrophy when they stumbled on a common gene in several modern strains of Prochlorococcus. The gene encoded the ability to break down chitin, a carbon-rich material that comes from the sloughed-off shells of arthropods, such as insects and crustaceans
“That was very strange,” says Capovilla, who decided to dig deeper into the finding when she joined the lab as a postdoc.
For the new study, Capovilla carried out experiments to see whether Prochlorococcus can in fact break down chitin in a useful way. Previous work in the lab showed that the chitin-degrading gene appeared in strains of Prochlorococcus that live in low-light conditions, and in Synechococcus. The gene was missing in Prochlorococcus inhabiting more sunlit regions.
In the lab, Capovilla introduced chitin particles into samples of low-light and high-light strains. She found that microbes containing the gene could degrade chitin, and of these, only low-light-adapted Prochlorococcus seemed to benefit from this breakdown, as they appeared to also grow faster as a result. The microbes could also stick to chitin flakes — a result that particularly interested Braakman, who studies the evolution of metabolic processes and the ways they have shaped the Earth’s ecology.
“People always ask me: How did these microbes colonize the early ocean?” he says. “And as Gio was doing these experiments, there was this ‘aha’ moment.”
Braakman wondered: Could this gene have been present in the ancestors of Prochlorococcus, in a way that allowed coastal microbes to attach to and feed on chitin, and ride the flakes out to sea?
It’s all in the timing
To test this new “chitin raft” hypothesis, the team looked to Fournier, who specializes in tracing genes across species of microbes through history. In 2019, Fournier’s lab established an evolutionary tree for those microbes that exhibit the chitin-degrading gene. From this tree, they noticed a trend: Microbes start using chitin only after arthropods become abundant in a particular ecosystem.
For the chitin raft hypothesis to hold, the gene would have to be present in ancestors of Prochlorococcus soon after arthropods began to colonize marine environments.
The team looked to the fossil record and found that aquatic species of arthropods became abundant in the early Paleozoic, about half a billion years ago. According to Fournier’s evolutionary tree, that also happens to be around the time that the chitin-degrading gene appears in common ancestors of Prochlorococcus and Synecococchus.
“The timing is quite solid,” Fournier says. “Marine systems were becoming flooded with this new type of organic carbon in the form of chitin, just as genes for using this carbon spread across all different types of microbes. And the movement of these chitin particles suddenly opened up the opportunity for microbes to really make it out to the open ocean.”
The appearance of chitin may have been especially beneficial for microbes living in low-light conditions, such as along the coastal seafloor, where ancient picocyanobacteria are thought to have lived. To these microbes, chitin would have been a much-needed source of energy, as well as a way out of their communal, coastal niche.
Braakman says that once out at sea, the rafting microbes were sturdy enough to develop other ocean-dwelling adaptations. Millions of years later, the organisms were then ready to “take the plunge” and evolve into the free-floating, photosynthesizing Prochlorococcus that exist today.
“In the end, this is about ecosystems evolving together,” Braakman says. “With these chitin rafts, both arthropods and cyanobacteria were able to expand into the open ocean. Ultimately, this helped to seed the rise of modern marine ecosystems.”
SignificanceAnd there we have a very nice illustration of how an environmental change (the presence of insects with their chitin exoskeletons) creates a new niche for an organism to move into, or in this case, onto. This takes them out into the ocean, where free-living becomes advantageous and they can then become independent of the chitin they were living on and take up a wholly photosynthesising existence. This newly abundant food resource then creates another opportunity for marine creatures to exploit.
The marine picocyanobacteria Prochlorococcus and Synechococcus are generally thought to be free-living planktonic cells. We show that some lineages can attach to, and use, particulate chitin, changing our view of their ecology. We also show that this trait was acquired around the time that arthropods, the main source of oceanic chitin, underwent a major ecological expansion. We propose the “chitin raft hypothesis”: Benthic ancestors of picocyanobacteria colonized the ocean alongside arthropods by attaching to free-floating exoskeleton particles. Co-expansion of both groups into the open ocean helped seed the rise of modern marine ecosystems. Loss of chitin utilization and transition to an exclusively planktonic lifestyle later occurred within a major subgroup of Prochlorococcus. It now numerically dominates the extant ocean.
Abstract
Marine picocyanobacteria Prochlorococcus and Synechococcus, the most abundant photosynthetic cells in the oceans, are generally thought to have a primarily single-celled and free-living lifestyle. However, while studying the ability of picocyanobacteria to supplement photosynthetic carbon fixation with the use of exogenous organic carbon, we found the widespread occurrence of genes for breaking down chitin, an abundant source of organic carbon that exists primarily as particles. We show that cells that encode a chitin degradation pathway display chitin degradation activity, attach to chitin particles, and show enhanced growth under low light conditions when exposed to chitosan, a partially deacetylated soluble form of chitin. Marine chitin is largely derived from arthropods, which underwent major diversifications 520 to 535 Mya, close to when marine picocyanobacteria are inferred to have appeared in the ocean. Phylogenetic analyses confirm that the chitin utilization trait was acquired at the root of marine picocyanobacteria. Together this leads us to postulate that attachment to chitin particles allowed benthic cyanobacteria to emulate their mat-based lifestyle in the water column, initiating their expansion into the open ocean, seeding the rise of modern marine ecosystems. Subsequently, transitioning to a constitutive planktonic life without chitin associations led to cellular and genomic streamlining along a major early branch within Prochlorococcus. Our work highlights how the emergence of associations between organisms from different trophic levels, and their coevolution, creates opportunities for colonizing new environments. In this view, the rise of ecological complexity and the expansion of the biosphere are deeply intertwined processes.
Capovilla, Giovanna; Braakman, Rogier; Fournier, Gregory P.; Hackl, Thomas, (2023)
Chitin utilization by marine picocyanobacteria and the evolution of a planktonic lifestyle, Proceedings of the National Academy of Sciences, 120(20), e2213271120 DOI: 10.1073/pnas.2213271120
Copyright: © 2023 The authors.
Published by PNAS.
Reprinted under a Creative Commons Attribution-NonCommercial-NoDerivatives License 4.0 (CC BY-NC-ND)
And so the delicate world-wide ecosystem comes to depend on a tiny, single-celled organism that we endanger at our peril.
And no magic or supernatural deities needed to explain it.
No comments :
Post a Comment
Obscene, threatening or obnoxious messages, preaching, abuse and spam will be removed, as will anything by known Internet trolls and stalkers, by known sock-puppet accounts and anything not connected with the post,
A claim made without evidence can be dismissed without evidence. Remember: your opinion is not an established fact unless corroborated.