Refuting Creationism
The Universe Is Not Fine-Tuned for Life. It is Fine-Tuned For Black Holes and Destruction
The Universe Is Not Fine-Tuned for Life. It is Fine-Tuned For Black Holes and Destruction
Supermassive black hole consuming a sun
Watch a star get destroyed by a supermassive black hole in the first simulation of its kind
Creationist have been fooled by their cult to believe that the Universe is 'fine-tuned' for life and that this 'fine-tuning' is proof of their particular god and its particular holy book.
This is a blatant false dichotomy fallacy; even if it could be proved to be true by showing that the various (and usually ill-defined) parameters could have other values it does not follow that a randomly designated god is responsible for 'tuning' them.
It's also a blasphemy, if creationists did but realise it, because it assumes their supposedly omnipotent god is itself constrained by the fundamental laws of chemistry and physics and could only work to create life within very narrow constraints - which implies a higher power to set that constraint.
How many black holes are there in the known Universe?But it is nonsensical for another reason: life is not the most abundant manifestation of the laws of physics in the Universe; that honour goes more deservedly to black holes, of which there are estimatedEstimating the exact number of black holes in the known Universe is challenging, but astronomers have made some educated guesses based on our current understanding.
Stellar-Mass Black Holes
Stellar-mass black holes, which form from the collapse of massive stars, are the most common type. Based on the number of stars in the Universe and the fraction of those stars that are massive enough to form black holes, it is estimated that there are around 100 million to 1 billion stellar-mass black holes in the Milky Way galaxy alone. Given that the Milky Way is just one of about 2 trillion galaxies in the observable Universe, the number of stellar-mass black holes across the Universe could be in the range ofto (a billion trillion to 10 billion trillion).
Supermassive Black Holes
Supermassive black holes, which exist at the centers of galaxies, are much rarer. Almost every galaxy with a significant size is thought to have a supermassive black hole at its center. This suggests there could be around 2 trillion supermassive black holes in the observable Universe, one for each galaxy.
Primordial Black Holes
There is also the theoretical possibility of primordial black holes, which might have formed in the early Universe. These could range in mass from very small to large and could number in the vast quantities, though their existence has not been confirmed.
Total Estimate
In total, combining these different types, the number of black holes in the observable Universe could be as high asor more. This estimate is still very rough, as it depends on many factors, including the formation rates of black holes, the life cycle of stars, and the evolution of galaxies.
And black holes, far from showing that the Universe is a place designed to be friendly to life (which only the most parochial and naive simpletons could imagine, given that most of it above a few thousand feet of the surface of Earth is hostile to most forms of life and only a fraction of the surface is hospitable without special adaption or complex technology), are about the most destructive thing in the Universe, reducing everything that comes within their event horizon to energy which can only escape in the form of Hawking radiation.
So, if anything, the Universe appears to be 'fine-tuned' for self-destruction and the eventual extermination of life. Not exactly what the creation cults want their dupes to believe.
This theory has the advantage of a possible explanation for the appearance of design just as living organisms have, in the form of the Theory of Evolution. Black holes are believed to contain the quantum conditions for universes to spontaneously arise, so, if there were a mechanism for passing information through a black hole from the parent universe to a descendant one, natural selection should mean universes get better at making black holes.
How black holes swallow up entire suns, compete with any orbiting planets is the subject of a recent paper in Astrophysical Journal Letters which show computer generated simulations of the event. One notable observation if the 'spaghettification' effect where, from the point of view of a distant observer, an object falling into a black hole becomes drawn out into a thin string, like toothpaste out of a tube. This is caused by the dilation of space and time due to the increasing gravity as the black hole is approached and is an effect of General Relativity.
One of the authors of this paper, Professor Daniel Price of Monash University, Australia, has published an article about the team's findings in The Conversation. His article is reprinted here under a Creative Commons license, reformatted for stylistic consistency:

Watch a star get destroyed by a supermassive black hole in the first simulation of its kind
Price et al. (2024)
Giant black holes in the centres of galaxies like our own Milky Way are known to occasionally munch on nearby stars.
This leads to a dramatic and complex process as the star plunging towards the supermassive black hole is spaghettified and torn to shreds. The resulting fireworks are known as a tidal disruption event.
In a new study published today in the Astrophysical Journal Letters, we have produced the most detailed simulations to date of how this process evolves over the span of a year.
A black hole tearing apart a sun
American astronomer Jack G. Hills and British astronomer Martin Rees first theorised about tidal disruption events in the 1970s and 80s. Rees’s theory predicted that half of the debris from the star would remain bound to the black hole, colliding with itself to form a hot, luminous swirl of matter known as an accretion disc. The disc would be so hot, it should radiate a copious amount of X-rays.
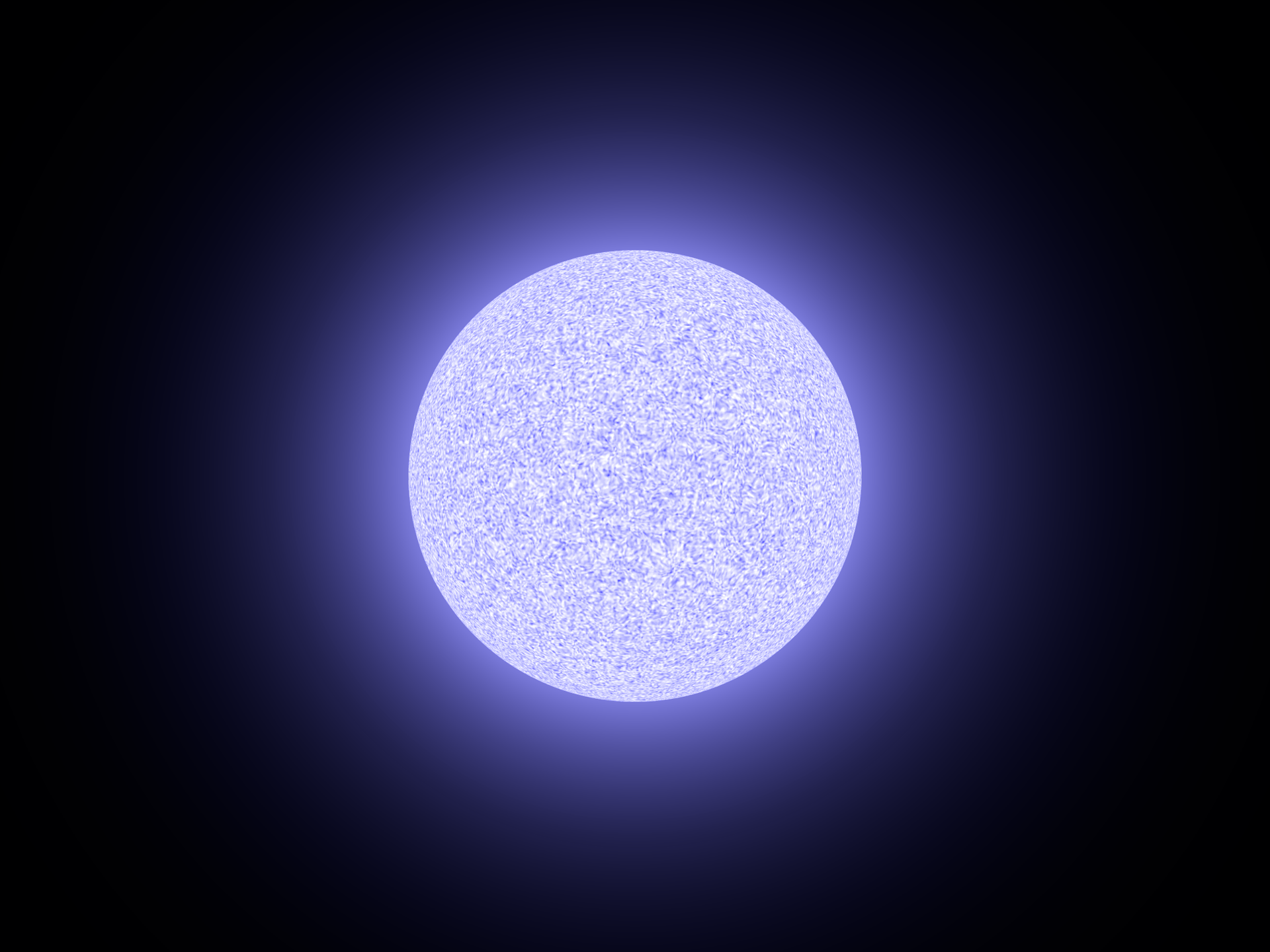
An artist’s impression of a moderately warm star – not at all what a black hole with a hot accretion disc would be like.
Even weirder is the inferred size of the glowing material around the black hole: several times larger than our Solar System and expanding rapidly away from the black hole at a few percent of the speed of light.
Given that even a million-solar-mass black hole is just a bit bigger than our Sun, the huge size of the glowing ball of material inferred from observations was a total surprise.
While astrophysicists have speculated the black hole must be somehow smothered by material during the disruption to explain the lack of X-ray emissions, to date nobody had been able to show how this actually occurs. This is where our simulations come in.
A slurp and a burp
Black holes are messy eaters – not unlike a five-year-old with a bowl of spaghetti. A star starts out as a compact body but gets spaghettified: stretched to a long, thin strand by the extreme tides of the black hole.
As half of the matter from the now-shredded star gets slurped towards the black hole, only 1% of it is actually swallowed. The rest ends up being blown away from the black hole in a sort of cosmic “burp”.
Simulating tidal disruption events with a computer is hard. Newton’s laws of gravity don’t work near a supermassive black hole, so one has to include all the weird and wonderful effects from Einstein’s general theory of relativity.
But hard work is what PhD students are for. Our recent graduate, David Liptai, developed a new do-it-Einstein’s-way simulation method which enabled the team to experiment by throwing unsuspecting stars in the general direction of the nearest black hole. You can even do it yourself.
Spaghettification in action, a close up of the half of the star that returns to the black hole.
They follow the spaghettification of the star through to when the debris falls back on the black hole, then a close approach that turns the stream into something like a wriggling garden hose. The simulation lasts for more than a year after the initial plunge.
It took more than a year to run on one of the most powerful supercomputers in Australia. The zoomed-out version goes like this:
Zoomed-out view, showing the debris from a star that mostly doesn’t go down the black hole and instead gets blown away in an expanding outflow.
To our great surprise, we found that the 1% of material that does drop to the black hole generates so much heat, it powers an extremely powerful and nearly spherical outflow. (A bit like that time you ate too much curry, and for much the same reason.)
The black hole simply can’t swallow all that much, so what it can’t swallow smothers the central engine and gets steadily flung away.
When observed like they would be by our telescopes, the simulations explain a lot. Turns out previous researchers were right about the smothering. It looks like this:
The same spaghettification as seen in the other movies, but as would be seen with an optical telescope [if we had a good-enough one]. It looks like a boiling bubble. We’ve called it the “Eddington envelope”.

Daniel Price, Professor of Astrophysics, Monash University
This article is republished from The Conversation under a Creative Commons license. Read the original article.
Any creationists wishing to refute this paper will need to refute the details given here:
AbstractThe Universe is far from the ideal environment for life to thrive in - known life only exists as an encrustation on or near the surface of a single planet. Instead, it is a violent and unstable chaos of competing forces with an estimated
Stars falling too close to massive black holes in the centres of galaxies can be torn apart by the strong tidal forces. Simulating the subsequent feeding of the black hole with disrupted material has proved challenging because of the range of timescales involved. Here we report a set of simulations that capture the relativistic disruption of the star, followed by one year of evolution of the returning debris stream. These reveal the formation of an expanding asymmetric bubble of material extending to hundreds of astronomical units — an outflowing Eddington envelope with an optically thick inner region. Such outflows have been hypothesised as the reprocessing layer needed to explain optical/UV emission in tidal disruption events, but never produced self-consistently in a simulation. Our model broadly matches the observed light curves with low temperatures, faint luminosities, and line widths of.
1 Introduction
In the classical picture of tidal disruption events (TDEs), the debris from the tidal disruption of a star on a parabolic orbit by a supermassive black hole (SMBH) rapidly circularises to form an accretion disc via relativistic apsidal precession (Rees, 1988). The predicted mass return rate of debris (Phinney, 1989) isand the light curve is assumed to be powered by accretion and to follow the same decay.
This picture alone does not predict several properties of observed TDEs, mainly related to their puzzling optical emission (van Velzen et al., 2011; van Velzen, 2018; van Velzen et al., 2021). These properties include: i) low peak bolometric luminosities (Chornock et al., 2014) of1 per cent of the value expected from radiatively efficient accretion (Svirski et al., 2017); ii) low temperatures, more consistent with the photosphere of a B-type star than with that of an accretion disc at a few tens of gravitational radii ( ) (Gezari et al., 2012; Miller, 2015), and consequently large emission radii, au for a black hole (Guillochon et al., 2014; Metzger & Stone, 2016); and iii) spectral line widths implying gas velocities of , much lower than expected from an accretion disc (Arcavi et al., 2014; Leloudas et al., 2019; Nicholl et al., 2019).
As a consequence, numerous authors have proposed alternative mechanisms for powering the TDE lightcurve, via either shocks from tidal stream collisions during disc formation (Lodato, 2012; Piran et al., 2015; Svirski et al., 2017; Ryu et al., 2023; Huang et al., 2023), or the reprocessing of photons through large scale optically thick layers, referred to as Eddington envelopes (Loeb & Ulmer, 1997), super-Eddington outflows (Strubbe & Quataert, 2009), quasi-static or cooling TDE envelopes (Roth et al., 2016; Coughlin & Begelman, 2014; Metzger, 2022) or mass-loaded outflows (Jiang et al., 2016; Metzger & Stone, 2016). Recent spectro-polarimetric observations suggest reprocessing in an outflowing, quasi-spherical envelope (Patra et al., 2022).
The wider problem is that few calculations exist that follow the debris from disruption to fallback for a parabolic orbit with the correct mass ratio. The challenge is to evolve a main-sequence star on a parabolic orbit around a SMBH from disruption and to follow the subsequent accretion of material (Metzger & Stone, 2016). The dynamic range involved when astar on a parabolic orbit is tidally disrupted by a SMBH is greater than four orders of magnitude: the tidal disruption radius is 50 times the gravitational radius, where general relativistic effects are important, while the apoapsis of even the most bound material is another factor of 200 further away. This challenge led previous studies to consider a variety of simplifications (Stone et al., 2019): i) reducing the mass ratio between the star and the black hole by considering intermediate mass black holes (Ramirez-Ruiz & Rosswog, 2009; Guillochon et al., 2014); ii) using a Newtonian gravitational potential (Evans & Kochanek, 1989; Rosswog et al., 2008; Lodato et al., 2009; Guillochon et al., 2009; Golightly et al., 2019), pseudo-Newtonian (Hayasaki et al., 2013; Bonnerot et al., 2016) or post-Newtonian approximations (Ayal et al., 2000; Hayasaki et al., 2016); iii) simulating only the first passage of the star (Evans & Kochanek, 1989; Laguna et al., 1993; Khokhlov et al., 1993; Frolov et al., 1994; Diener et al., 1997; Kobayashi et al., 2004; Guillochon et al., 2009; Guillochon & Ramirez-Ruiz, 2013; Tejeda et al., 2017; Gafton & Rosswog, 2019; Goicovic et al., 2019); and iv) assuming stars initially on bound, highly eccentric orbits instead of parabolic orbits (Sadowski et al., 2016; Hayasaki et al., 2013, 2016; Bonnerot et al., 2016; Liptai et al., 2019; Hu et al., 2024).
These studies have, nevertheless, provided useful insights into the details of the tidal disruption process. In particular, it has been shown that the distribution of orbital energies of the debris following the initial disruption is roughly consistent with= const, consistent with the analytic prediction of a mass fallback rate, although the details can depend on many factors such as stellar spin, stellar composition, penetration factor and black hole spin (Lodato et al., 2009; Kesden, 2012; Guillochon & Ramirez-Ruiz, 2013; Golightly et al., 2019; Sacchi & Lodato, 2019). The importance of general relativistic effects in circularising debris has also been demonstrated. The self-intersection of the debris stream, which efficiently dissipates large amounts of orbital energy, is made possible by relativistic apsidal precession (Hayasaki et al., 2016; Bonnerot et al., 2016; Liptai et al., 2019; Calderón et al., 2024). But until recently debris circularisation has only been shown for stars on bound orbits, with correspondingly small apoapsis distances and often deep penetration factors (we define the penetration factor as , where is the tidal radius and is the pericenter distance).
Recent works have shown that circularisation and initiation of accretion is possible in the parabolic case, by a combination of energy dissipation in the ‘nozzle shock’ that occurs on second pericenter passage (Steinberg & Stone 2024; but see Bonnerot & Lu 2022 and Appendix E for convergence studies of the nozzle shock) and/or relativistic precession leading to stream collisions (Andalman et al., 2022). In this paper, we present a set of simulations that self-consistently evolve a one solar mass polytropic star on a parabolic orbit around asolar mass black hole from the star’s disruption to circularization of the returning debris and then accretion. We follow the debris evolution for one year post-disruption, enabling us to approximately compute synthetic light curves which appear to match the key features of observations.
Figure 1:One year in the life of a tidal disruption event. We show shapshots of column density in the simulation of astar on a parabolic orbit with , disrupted by a black hole, using SPH particles in the Schwarzschild metric. Main panel shows the large scale outflows after 365 days projected in the plane with log scale. Inset panels show the stream evolution on small scales ( au), showing snapshots of column density projected in the plane on a linear scale from (colours are allowed to saturate). Animated versions of this figure are available in the online article. Data and scripts used to create the figure are available on Zenodo:https://doi.org/10.5281/zenodo.11438154 (catalog doi:10.5281/zenodo.11438154) Price, Daniel J.; Liptai, D.; Mandel, I; et al (2024)
Eddington envelopes: The fate of stars on parabolic orbits tidally disrupted by supermassive black holes
arXiv:2404.09381v2 [astro-ph.HE] DOI: 10.48550/arXiv.2404.09381
Copyright: © [year] The authors.
Published by [publisher]. Open access.
Reprinted under a Creative Commons Attribution-ShareAlike 4.0 International (CC BY-SA 4.0)
It requires parochial ignorance of the first order to imagine that the entire Universe is designed for life. It is far easier to make a case for it being designed for black holes, although that, as with the 'designed for life' case, case would require a priori evidence of the existence of a creative entity in the form of an explanation of its origins and definitive evidence of it ever being recorded as creating anything. And by recorded, I don't mean written in the mythology of Bronze Age pastoralists who thought the Universe was a small flat place with a dome over it and containing nothing that was unknown within a day or two's walk of the Canaanite Hills where they grazed their goats, and later decreed to be literal history by people with a vested interest in people believing the myths.
What Makes You So Special? From The Big Bang To You
How did you come to be here, now? This books takes you from the Big Bang to the evolution of modern humans and the history of human cultures, showing that science is an adventure of discovery and a source of limitless wonder, giving us richer and more rewarding appreciation of the phenomenal privilege of merely being alive and able to begin to understand it all.
Available in Hardcover, Paperback or ebook for Kindle
Ten Reasons To Lose Faith: And Why You Are Better Off Without It
This book explains why faith is a fallacy and serves no useful purpose other than providing an excuse for pretending to know things that are unknown. It also explains how losing faith liberates former sufferers from fear, delusion and the control of others, freeing them to see the world in a different light, to recognise the injustices that religions cause and to accept people for who they are, not which group they happened to be born in. A society based on atheist, Humanist principles would be a less divided, more inclusive, more peaceful society and one more appreciative of the one opportunity that life gives us to enjoy and wonder at the world we live in.
Available in Hardcover, Paperback or ebook for Kindle
Last Modified: Sun Mar 30 2025 22:10:32 GMT+0000 (Coordinated Universal Time)
No comments :
Post a Comment
Obscene, threatening or obnoxious messages, preaching, abuse and spam will be removed, as will anything by known Internet trolls and stalkers, by known sock-puppet accounts and anything not connected with the post,
A claim made without evidence can be dismissed without evidence. Remember: your opinion is not an established fact unless corroborated.